Neural Circuitry
- By Roger Brooking
- Mar 9, 2015
- 7 min read
The stretch shortening cycle and the mechanism of counter-movement jumps
Counter-movement jumps (CMJ) are performed in many sports and the main mechanism of this type of contraction is the stretch shortening cycle (SSC). The stretch shortening cycle combines the elastic properties of tendons, connective tissue as well as the stretch reflex (Tortora & Derrickson, 2012). Also, at a deeper level the SSC relies on the integration of the sensory systems such as proprioceptive, visual and vestibular systems. These systems keep the athlete in balance, give him a sense of direction and enhance jumping performance. The SSC is known to potentiate 15%-20% of the concentric phase of the counter movement (Cardinale, Newton & Nosaka, 2011).
The stretch reflex - The monosynaptic reflex is the simplest form of the stretch reflex. When a muscle is stretched, type 1a sensory afferent fibers are triggered by the lengthening of the muscle spindle in the intrafusal muscles fibre. This stretch stimulates a signal that generates a nerve impulse on the 1a afferent fibers, which travels toward the spinal cord and synapses with an efferent α-motorneuron. The α-motorneuron sends the impulse back to the same muscle for the extrafusal muscle fibers which counteract the stretch activating large muscle fibers concentrically (Courtine, Harkema, Dy, Gerasimenko, & Dyhre-Poulsen, 2007). In addition to this, the antagonist muscle needs to relax in order for agonist muscle to contract. This reflex is called reciprocal inhibition, and originates from the same type 1a afferent. This now travels to an inhibitory interneuron on the spinal cord which synapses with an α-motorneuron from the antagonist muscle, decreasing the activation of the muscle (Crewther, Cook, Cardinale, & Weatherby, 2011). This double synapse is called polysynaptic reflex and one example of this reflex is the quadriceps tendon test in the picture below. This creates a stretch in the quadriceps that stimulates the muscle spindles making the quadriceps contract and the hamstrings relax in order for movement to occur.
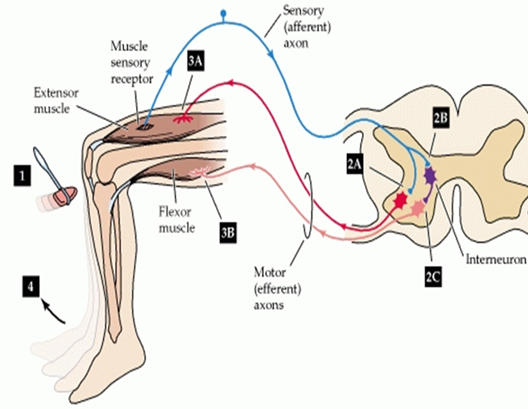
(Adapted from Tortora et al, 2012)
Golgi tendon organs – The Golgi tendon organs (GTOs) are responsible for the control of excessive force on the agonist muscle which protects the tendon and muscle from excessive stress. This reflex is called autogenic inhibition and the neural conductivity originates form GTOs attached to the tendon. The GTOs synapse to a type Ib sensory afferent and are stimulated when the tendon is stretched. This sends a signal towards the spinal cord to connect to an inhibitory interneuron which synapses to an α-motorneuron (Baechle & Earle, 2008). The signal inhibits the α-motorneuron from the agonist muscle which decreases the force in order to avoid injury. Training adaptations lead the nervous system to decrease GTOs sensibility, which will eventually increase the level of strength (Chalmers, 2002).
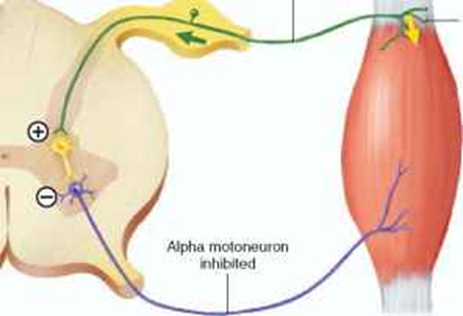
Example of autogenic inhibition
Recurrent inhibition - Recurrent inhibition is a basic type of neuronal circuit throughout the central nervous system. In the spinal cord, motoneurons give off axon collaterals to excite GABAergic/glycinergic Renshaw cells, which mediate recurrent inhibition of motoneurons, Ia inhibitory interneurons, Renshaw cells and some cells of origin of the ventral spinocerebellar tract. Since the Renshaw cells also inhibit Ia inhibitory interneurons, which inhibit antagonistic motoneurons, activation of the Renshaw cells leads to removal of the inhibition of the antagonist motoneurons, and this phenomenon is termed recurrent facilitation. The Renshaw cells are located near the cell bodies of the alpha motoneurons in the spinal cord. When the alpha motoneurons are excited and send signals to the muscle fibers to contract, the Renshaw cells are also excited. These Renshaw cells make inhibitory connections on these same alpha motoneurons, thus preventing them from sending more signals down to the muscle fibers.
Proprioceptors – These are responsible for sensory feedback between the central nervous system, muscles, tendons and joints. They are fundamental in controlling body movements providing information for balance, coordination, muscle length tension, posture and equilibrium (Gurney, Milani, Pedersen, Elizabeth, & Role, 2000).
Series of Elastic Components (SEC) – The mechanism is responsible for collecting elastic energy and this energy is stored in the tendons and soft tissues around the muscles. The SEC works synergistically with the parallel elastic components (PEC) which are non-contractile elastic proteins that run parallel within the muscle fiber (Alter, 2004; Radcliffe & Farentinos, 1999). When a muscle undertakes an eccentric action, the SEC act like a spring, accumulating elastic energy at the end of the deceleration phase. This energy creates a recoil effect which gives energy back, potentiating the concentric contraction (Baechle et al., 2008).
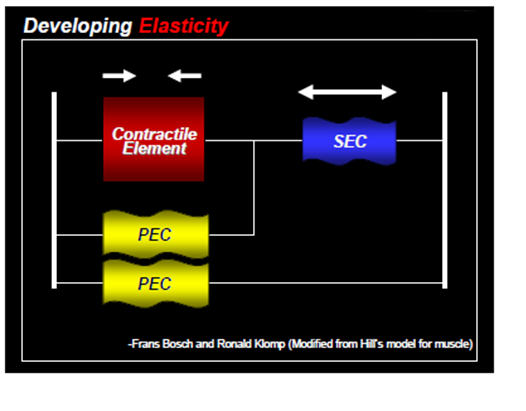
Coactivation – Sometimes muscles needs to work synergistically to stabilize the joint. This type of contraction is when the antagonist muscle contracts as well in order to maintain joint stability with the assistance of the proprioceptors. All muscles work together to fine tune joint control.
The Stretch Shortening Cycle Phases
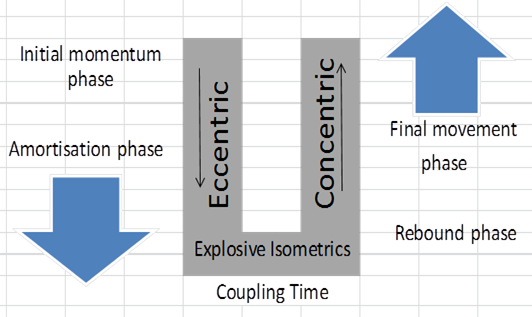
(Adapted from Supertraining- PG, 270)
Initial momentum Phase – This phase happens in a CMJ when an athlete is coming down from a previous jump such as a drop jump (Verkhoshansky et al., 2008). The first contact on landing lengthens the muscles and triggers the muscle spindle afferent fibers to discharge a signal that creates a polysynaptic response. Research shows that the frequency of the afferent signal is modulated by the intensity of the eccentric phase which helps to decelerate the limb progressively (Dyhre-Poulsen, Simonsen & Voigt, 1991). As the eccentric force increases, so do the afferent and efferent signals, thus working like a controlled suspension. In addition, during the eccentric phase, muscles start to gather elastic energy and stored energy occurs from one segment to the next via kinetic linkage generating great torques of elastic energy within the kinetic chain (Verkhoshansky et al., 2008).
Amortization Phase – This phase is where the stretch reflex has a higher response and counteracts the descending movement with greater eccentric contraction followed by an explosive isometric contraction. While the isometric contraction happens, the SEC is elongated similar to lengthening a spring.
Coupling Time - Coupling time is the transition time between the eccentric and concentric phases of the SSC. Short coupling times are thought to be related to a more efficient utilization of elastic energy in the SSC. Similarly, short contact times are also important for maximizing the potentiating effects of the SSC, as delay in coupling time has shown a decrease in vertical jumps (Komi, 2003).

Rebound Phase – This phase is where an athlete moves upwards releasing all the energy from the SEC, working synergistically with both the stretch reflex and voluntary concentric action.
Final Movement Phase – During this phase an athlete continues to use the concentric energy potentiate by the stretch reflex and SEC, and starts to push off the ground moving towards triple extension (Baechle et al., 2008).
Fatigue - Komi (2000) has shown that the SSC fatigues occur due to the overuse of the muscle spindles and tearing of elastic mechanism inside the spindles (Tintin). This deterioration of structural proteins reduces afferent feedback inhibiting the stretch reflex and decreasing jump power output.
Training Considerations
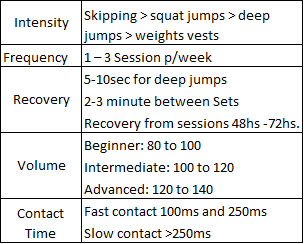
(Adapted from Baechle & Earle, 2008; McClymont & Hore, 2003)
Bottom line – Improvements in the SSC can lead to a quick neural response, enhancing neural signaling and, therefore, making an athlete faster (Gruber & Gollhofer, 2004). Therefore, improving the mechanism of SSC leads to enhancements in dynamic stabilization, performance, explosive power, reaction time and decreases potential for injury (Gollhofer, 2000).
Referencing
Buresh, R., Berg, & French. (2009). The Effect of Resistive Exercise Rest Interval on Hormonal Response, Strength, and Hypertrophy with Training. Journal of Strength & Conditioning Research (Allen Press Publishing Services Inc.), 23(1), 62-71.
Cadore, E. L., Lhullier, F. L. R., Brentano, M. A., Da Silva, E. M., Ambrosini, M. B., Spinelli, R., Silva, R. F., et al. (2008). Hormonal responses to resistance exercise in long-term trained and untrained middle-aged men. The Journal of Strength & Conditioning Research, 22(5), 1617-1624.
Crewther, B. T., Cook, C., Cardinale, M., & Weatherby, R. P. (2011). Two Emerging Concepts for Elite Athletes Neuromuscular System and the Dose-Response Training Role of these Endogenous Hormones. Sports Medicine, 41(2), 103-123.
Kraemer, W. J., Dunn-Lewis, C., Comstock, B. a, Thomas, G. a, Clark, J. E., & Nindl, B. C. (2010). Growth hormone, exercise, and athletic performance: a continued evolution of complexity. Current sports medicine reports, 9(4), 242-52.
West, D. W. D., & Phillips, S. M. (2010). Anabolic processes in human skeletal muscle: restoring the identities of growth hormone and testosterone. The Physician and sportsmedicine, 38(3), 97-104.
Alter, M (2004). Science of Flexibility. 3rd ed. USA: Champaign - Human Kinetics. (Pp. 53 -71).
Baechle, T. R., Earle, R. W. & Wathen, D. (2008). Resistance Training .In: T. R. Baechle, T. & R. W. Earle, Essentials of Strength Training and Conditioning. 3rd ed. (Pp. 381-412) Honk Kong: Human Kinetics.
Cardinale, M., Newton, R. & Nosaka, K. (Eds), Strength and Conditioning Biological Principles and Practical Applications. UK: John Wiley & Sons, Ltd. 223-235.
Chalmers, G. (2002). Do Golgi tendon organs really inhibit muscle activity at high force levels to save muscles from injury, and adapt with strength training? Sports biomechanics International Society of Biomechanics in Sports, 1(2), 239-249.
Courtine, G., Harkema, S. J., Dy, C. J., Gerasimenko, Y. P., & Dyhre-Poulsen, P. (2007). Modulation of multisegmental monosynaptic responses in a variety of leg muscles during walking and running in humans. The Journal of physiology, 582(Pt. 3), 1125-1139.
Dyhre-Poulsen, P., Simonsen, E. B. & Voigt, M. (1991). Dynamic control of muscle stiffness and H reflex modulation during hopping and jumping in man. The Journal of Physiology, 437, 287-304.
Gollhofer, A. (2000). Importance of proprioceptive activation on functional neuromuscular properties. Available: http://w4.ub.uni-konstanz.de/cpa/article/view/2294. Last accessed 14/02/2012.
Gruber, M., & Gollhofer, A. (2004). Impact of sensorimotor training on the rate of force development and neural activation. European Journal of Applied Physiology, 92, 98-105.
Gurney, B., Milani, J., Pedersen, M. E., Elizabeth, M., & Role, P. (2000). Role of fatigue on proprioception of the ankle. Journal of Exercise Physiology online, 3(1), 8-14.
Komi, P, V. (2003). Stretch–Shortening Cycle. In: Komi, P, V. Strength and power in sport. 2nd ed. UK: Blackwell Science. 184 - 202.
Komi, P. V. (2000). Stretch-shortening cycle: a powerful model to study normal and fatigued muscle. Journal of Biomechanics, 33, 1197-1206.
McClymont, D. and Hore, A. (2003). Use of the Reactive Strength Index (RSI) as a plyometric monitoring tool. Available: http://www.coachesinfo.com/index.php?option=com_docman&task=cat_view&gid=45&Itemid=76. Last accessed 15/02/2012.
Radcliffe, J, C. & Farentinos, B, C. (1999). High-powered plyometrics. USA: Human Kinetics. 1 - 38.
Tortora, G, J. & Derrickson, B. (2012). Sensory, motor and integrative systems In: G, J, Tortora. & B, Derrickson. Principles of Anatomy & Physiology. 13th ed. USA: John Wiley & Sons, Inc. 606 - 634.
Verkhoshansky, Y. & Siff, M. (2009). Supertraining. 6th ed. Ultimate Athletes Concept. Pp176 -
Comments